Shapeshifting Robotic Microswarm: Revolutionizing Oral Care
In recent years, groundbreaking innovations have reshaped the landscape of healthcare technology, and one such innovation promises to transform the way we approach oral care. A multidisciplinary team at the University of Pennsylvania has pioneered a remarkable technology that has the potential to revolutionize the mundanity of daily oral hygiene. This revolutionary system comprises shapeshifting robotic microswarms, powered by iron oxide nanoparticles, offering an automated solution to brushing, rinsing, and flossing, all in one seamless process.
The Iron Oxide Nanoparticles
At the core of this groundbreaking technology are iron oxide nanoparticles with dual functionalities: catalytic and magnetic. These nanoparticles possess the unique ability to respond to magnetic fields, enabling researchers to control their motion and formation. They can be programmed to shape themselves into bristle-like structures or elongated strings, capable of reaching every nook and cranny in the oral cavity.
Combatting Harmful Oral Bacteria
One of the key advantages of this robotic system is its ability to combat harmful oral bacteria effectively. When the microrobots are in action, a catalytic reaction is triggered within the iron oxide nanoparticles. This reaction produces antimicrobials on-site, swiftly annihilating the oral bacteria responsible for cavities and gum diseases. The result? A comprehensive and precise cleaning process that significantly reduces the risk of dental issues.
Versatile and Adaptive
Experiments with this innovative system have demonstrated its remarkable versatility and adaptability. Whether a patient has straight teeth or misaligned ones, the microrobots conform effortlessly to different surfaces. They can seamlessly adjust to the unique topographies of an individual’s oral cavity, ensuring a thorough and personalized cleaning experience.
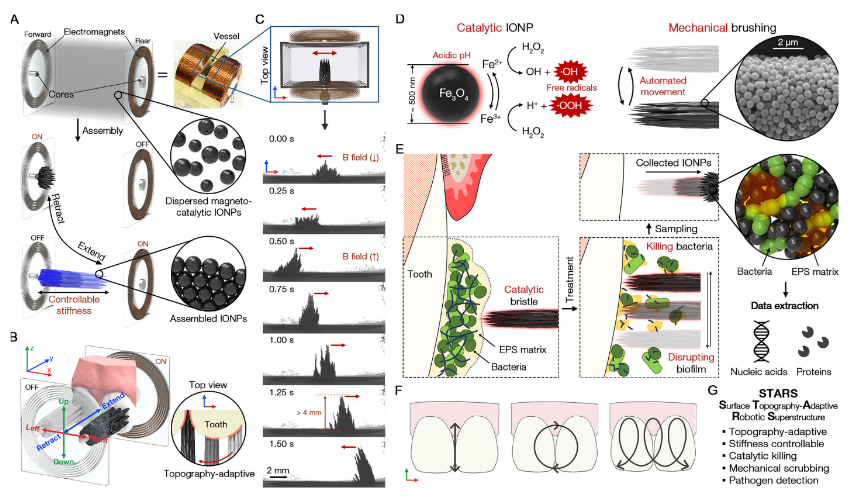
Assembly, control, and functional properties of STARS. (A) IONPs are dispersed in solution between two electromagnets. IONPs are collected on the forward wall of the vessel, then extended into a bristle-like superstructure with controllable stiffness. (B) The forward electromagnet core guides the bristles across the target surface with topography-adaptive property. (C) (top-view) IONPs (1 mg mL–1) are initially collected in a low aspect ratio mound and then extended into a high aspect ratio bristle-like formation as they are swept laterally. (D) IONPs are multifunctional with peroxidase-like activity, generating free radicals at the site of mechanical cleaning providing both antimicrobial treatment and physical biofilm removal. (E) Bristle motion is controlled to disrupt biofilms through mechanochemical action and retrieve biofilm contents (microbes, extracellular polysaccharides, biomolecules) for diagnostic sampling. (F) Programmable motions enable targeted treatment and optimized cleaning via automated routines. (G) Multifunctional and multitasking capabilities integrated into STARS.
Disrupting the Status Quo
For centuries, the design of the toothbrush has remained relatively unchanged. While incremental improvements have been made, the fundamental concept of a ‘bristle-on-a-stick’ has endured. This revolutionary technology disrupts the dental care industry by introducing a level of automation and efficiency never seen before.
A Synergy of Research
The development of this transformative technology is a result of the synergy between two distinct research groups at the University of Pennsylvania. Researchers from the School of Dental Medicine and the School of Engineering and Applied Science came together, each with their unique interests in iron oxide nanoparticles. The dental team was intrigued by their catalytic properties, while the engineering team was exploring their potential in magnetically controlled microrobots. This collaboration ultimately led to the creation of a platform that could electromagnetically control the microrobots and deliver antimicrobials precisely where needed.
Proven Effectiveness
Extensive testing has been conducted to validate the effectiveness of this robotic system. Microrobots were tested on various surfaces, including 3D-printed tooth models based on real human teeth and real human teeth themselves. In all cases, the microrobots exhibited their remarkable ability to eliminate biofilms, eradicating pathogens and preventing dental issues. Notably, the iron oxide nanoparticles used have already been FDA approved for other applications, and tests on animal models have shown that the bristle formations do not harm gum tissue.
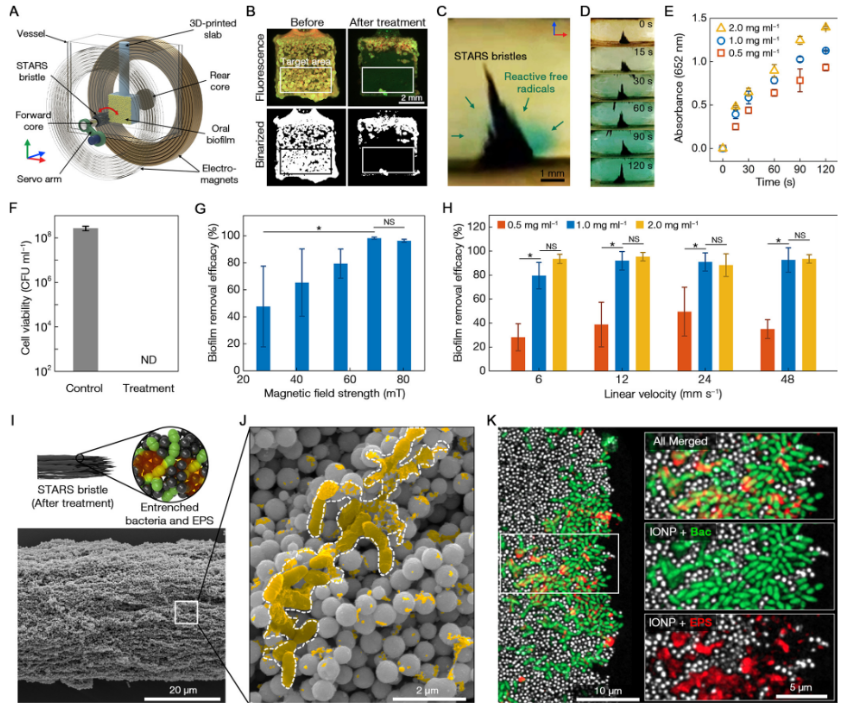
Biofilm removal, bacterial killing, and retrieval of biofilm components using STARS. (A) A schematic of the experimental platform for measuring efficacy of biofilm cleaning. Physical dimension (width × depth × height) of the slab is 4.0 × 2.2 × 4.0 mm3. (B) Biofilm cleaning efficacy is evaluated across the targeted area (4.0 × 2.0 mm2) using fluorescent labeling and binarized image analysis. (C–E) Catalytic activity in situ. TMB assays demonstrate the generation of ROS on-site from H2O2 by the catalytically active (peroxidase-like) STARS bristles (1 mg mL–1, 12 mm s–1). (F) Cell viability counts show complete killing of targeted biofilms via catalytic activation of H2O2. ND means not detectable. (G) Biofilm removal efficacy increases as magnetic field and bristle stiffness increase. Data are mean ± SD, *P = 0.0249; NS means not significant P > 0.9999 (n = 3). (H) Biofilm removal efficacy increases with IONP concentration and is correlated with scrubbing velocity. (I) Biofilm components (microbial cells and extracellular material) become entrenched among the assembled STARS bristles. (J, K) SEM and confocal images demonstrate the entrenchment and retrieval of biofilm components, that is, bacteria (Bac) and extracellular polysaccharides (EPS). Data are mean ± SD, *P < 0.0001; NS means not significant P > 0.05 (n = 4); one-way ANOVA followed by post hoc Tukey’s test.
Customizable and Patient-Centric
The true power of this robotic oral care system lies in its customizability. Researchers have fine-tuned the motions of the microrobots, allowing for precise control of bristle stiffness and length. This level of customization ensures that the system is not only effective but also gentle enough for clinical use. It can be tailored to accommodate the specific needs of each patient, making it an invaluable tool for improving oral health.
The Path Forward
As this groundbreaking technology continues to evolve, the team at the University of Pennsylvania is dedicated to optimizing the robots’ motions and exploring different methods of delivering the microrobots through mouth-fitting devices. The ultimate goal is to make this innovative device accessible to a wide range of individuals, including the geriatric population and those with disabilities. With the potential to disrupt conventional oral care modalities, this technology is poised to advance oral healthcare to new heights.
In conclusion, the development of shapeshifting robotic microswarms powered by iron oxide nanoparticles represents a quantum leap in the field of oral care. Its automated, customizable, and precise cleaning capabilities have the potential to transform the way we maintain our oral health. This innovative technology not only disrupts the status quo but also holds the promise of significantly improving oral health outcomes for diverse populations.
For a visual representation of how this technology works, consider the following diagram in Markdown Mermaid syntax:
This diagram illustrates the key components and benefits of the shapeshifting robotic microswarm technology, highlighting its potential to revolutionize oral healthcare.
Sources
- Science Daily – Shapeshifting microrobots can brush and floss teeth – 5 July 2022.
- ACS Publications – Surface Topography-Adaptive Robotic Superstructures for Biofilm Removal and Pathogen Detection on Human Teeth – 28 June 2022.